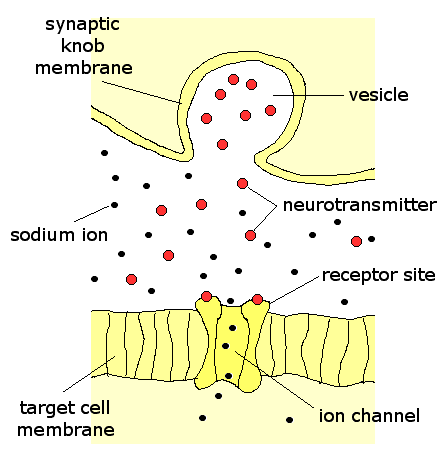
From Sandra Ackerman's book "Discovering the Brain": To be recognized as a neurotransmitter, a chemical compound must satisfy six conditions: It must be
OK, well, what about hormones? They're chemical messengers too - how are hormones different from neurotransmitters? A hormone, by definition, is a compound produced by an endocrine gland and released into the bloodstream where it can find it's target cells at some distance from it's actual site of release. A neurotransmitter on the other hand is a compound released from a nerve terminal.
When an electrical impulse travels to the end of a nerve cell, it stimulates the terminal of this cell to secrete a chemical signalling molecule at a special junction between nerve cells called a synapse. These nerve terminals are in direct apposition with their target cells to ensure rapid and specific delivery of the signal. This mode of transmission is in general much faster than the endocrine transmission mentioned above.
Both target cells possess receptors for the signalling molecule and may produce identical biochemical responses, it's just a question of the release mechanism that determines whether or not a given molecule is a neurotransmitter or a hormone. So, in the case of adrenaline, it's a hormone when the adrenal gland releases it into the bloodstream and it goes to the heart or the lungs OR it's a neurotransmitter when it is released from a stimulated presynaptic nerve cell and acts on it's neighbouring postsynaptic cell. (ref.).
From Samuel Barondes essay: "Drugs, DNA and the Analyst’s Couch" in “The Next Fifty Years: Science in the first half of the twenty-first century” (Good book! Recommended.)
In 1950, a chemist at Rhône-Poulenc, a French pharmaceutical company, modified the structure of an antihistamine and accidentally created a drug that can eliminate the psychotic thinking of people with schizophrenia. Within a few years the drug became world famous as chlorpromazine (Thorazine), the first truly effective medication for a disabling mental disorder. Because of its dramatic effect, chlorpromazine set a new course for psychiatry for the rest of the twentieth century. (Up to that time, one of the 'recommended' treatments for schizophrenia was lobotomy)
The great success of chlorpromazine stimulated vigorous competition from other pharmaceutical companies. In the 1950s, the search for more antipsychotic medications led to the accidental discovery of two other types of phsychiatric drugs. First Geigy, a Swiss pharmaceutical company, came up with a modified version of one of its antihistamines that, although useless against psychosis, proved to be a valuable treatment for severe depression. Named imipramine (Tofranil), it paved the way for many contemporary antidepressants. Then Hoffman-La Roche, another Swiss company, created chlordiazepoxide (Librium), which doesn’t help psychosis either but does relieve anxiety. It was soon followed by another benzodiazepine, diazepam (Valium), which became the best-selling drug in America for about a decade, beginning in the mid-1960s.
Adding to the excitement over these drugs were a flurry of findings about their effects on neurotransmitters, a class of brain chemicals that transmit signals between nerve cells. By the 1970s it was discovered that chlorpromazine blocks certain actions of a neurotransmitter called dopamine; imipramine augments the actions of several neurotransmitters, including norephinephrine and serotonin; and diazepam amplifies the effects of yet another neurotransmitter, called gamma-aminobutyric acid [GABA]. In each case, the net result is a change in signaling in brain circuits that control emotional aspects of behaviour.
These discoveries spurred a search for other chemicals that would have similar effects on neurotransmission but fewer undesirable side effects than the originals. The search paid off in a stream of new medications that patients perfer. The most famous, fluoxetine [Prozac], was initially identified as a chemical that prolongs neurotransmission by serotonin; it was subsequently shown to be an effective treatment for both severe and moderate depression. Called an SSRI (selective serotonin reuptake inhibitor), it prolongs serotonin’s effects by inhibiting its reuptake by the nerves that release it, which is the normal way that serortonin signaling is terminated. Related drugs, including sertraline (Zoloft), paroxetine (Paxil), fluvoxamine (Luvox), and citalopram (Celexa) soon followed.
So, drugs that affect neurotransmitters are big business. But all is not rosy with this picture: e.g. Seniors and Drugs: Prescribed to death - CBC news, Atypical antipsychotic drugs and risk of ischaemic stroke - British Medical Journal.
Neutrotransmitters
Found a number of fascinating stories of how neurotransmitters were discovered and sketches of what these things do.
Acetylcholine:
One of the first neurotransmitters found was acetylcholine (ACh). Widespread throughout the central nervous system, ACh usually has an excitatory function, but it can also be inhibitory, depending on prevailing conditions at the receptor site. Because ACh acts briskly and is subject to prompt breakdown in the synaptic cleft, it is well suited as the transmitter for motor neurons. Acetylcholine also acts in the autonomic nervous system, where it is responsible for such functions as contracting the pupil of the eye, slowing heartbeat, and stimulating salivation and digestion.
Two poisons that are well known to readers of mystery novels work their deadly effects by blocking the action of ACh. Curare, a plant extract used by South American Indians to treat their arrows for hunting, rapidly causes paralysis; botulin, a toxin produced by bacteria in improperly canned foods, paralyzes the muscles that control breathing and thereby causes suffocation.
In clinical practice, drugs that block the action of ACh are useful in many ways. Short-lived ACh inhibitors are given in eye drops to dilate the pupil for ophthalmic examination. More enduring forms, such as atropine, reduce the secretion of saliva and bronchial fluid, which is helpful for anesthesia; hyoscine, or scopolamine, another related compound, is sometimes used as a sedative but does have the side effect of causing a very dry mouth. Conversely, drugs that inhibit the chemical breakdown of ACh and thus extend its action in the synaptic cleft are at least temporarily effective against myasthenia gravis; this is a crippling disease in which the body's own immune system attacks the receptor sites for ACh on the skeletal muscles. The muscles gradually weaken as they receive fewer synaptic transmissions, but drugs such as eserine can effectively increase the amount of ACh available to the remaining receptor sites.
In the cerebral cortex, ACh is thought to play a role in storing short-term memories. The hippocampus, for example, has dense areas of receptor sites for ACh. (ref.)
Some of the receptors that ACh binds to are also bound to by nicotine.
Serotonin: a powerful constrictor of blood flow and an inhibitor of some sensations of pain; in recent years, its intriguing variety of effects on our mental life have also come under study. Serotonin is of great importance in regulating sleep; the old folk remedy for sleeplessness, a glass of warm milk before bedtime, may work because of the presence in milk of tryptophan, an amino acid that the brain uses to make serotonin. This transmitter can affect many parts of the brain at once through the long-reaching axons of serotonergic (serotonin-using) neurons, which underlie the transmitter's role in such global phenomena as sleep and mood. The drugs that raise levels of available norepinephrine to alleviate depression also work on serotonin, by the same mechanisms.
(Interestingly, the axons that carry serotonin are not myelinated. Without the electrical insulation afforded by the myelin sheath, impulses travel at less than the lightning speed achieved by, say, signals to the motor neurons, but this seems appropriate to the more global and subjective areas of life regulated by serotonin.) The remarkable effects of lysergic acid diethylamide, or LSD, in even the tiniest quantities, are based on its strong chemical resemblance to serotonin; it is as if a full system of preexisting receptor sites lies ready for the drug's use. One further aspect of this versatile transmitter is that serotonin is featured in biochemical accounts of “sensitization,” the enhanced response to a stimulus as a result of training. Scientists have studied sensitization in extraordinary detail in simple animals such as the marine snail as a model for more complex processes of learning in the human brain (ref.)
Drugs that binds to the serotonin receptors? LSD and Psilocybin
GABA: often acts as a fast synaptic transmission inhibitor. Unlike dopamine or serotonin, which have diverse roles, GABA consistently acts as an “off” signal; the cerebellum, retina, and spinal cord all use this transmitter to inhibit signals, as do many other parts of the brain and nervous system. GABA's inhibitory effect comes about in the following way: the transmitter opens a channel in the membrane through which negatively charged chloride ions can enter the cell. This influx hyperpolarizes the cell and makes it less likely to be excited by incoming stimuli. GABA receptor sites show some tendency to bind barbiturates and the “minor tranquilizers,” the benzodiazepines. Curiously, the presence of GABA in low concentrations enhances the binding of benzodiazepines to receptor sites. This pattern indicates that GABA and the benzodiazepines cannot be competing for exactly the same sites. Instead, an array of recent studies have yielded the view that the GABA receptor site is in fact a multifunctional set of proteins that contain the chloride ion channel and distinct subsites for binding of benzodiazepines, other tranquilizers such as barbiturates, and GABA itself. (ref.)
It appears that both benzodiazepine and barbiturates act by enhancing neural inhibition in the GABA system, although they act at different receptor sites. (ref.)
Glycine: Glycine is one of the basic amino acids that are used to build protein molecules. Glycine's function as a neurotransmitter is also fairly simple. When released into a synapse, glycine binds to a receptor which makes the post-synaptic membrane more permeable to Cl- ion. This hyperpolarizes the membrane, making it less likely to depolarize. Thus, glycine is an inhibitory neurotransmitter. It is de-activated in the synapse by a simple process of reabsorption by active transport back into the pre-synaptic membrane.
Glycine is a neurotransmitter only in vertebrate animals. The glycine receptor is primarily found in the ventral spinal cord. Strychnine is a glycine antagonist which can bind to the glycine receptor without opening the chloride ion-channel (ie, it inhibits inhibition). (ref.)Aspartate:
Like glycine, apartate is primarily localized to the ventral spinal cord. Like glycine, aspartate opens an ion-channel and is inactivated by reabsorption into the pre-synaptic membrane. Unlike glycine, however, apartate is an excitatory neurotransmitter, which increases the likelihood of depolarization in the postsynaptic membrane. Aspartate & glycine form an excitatory/inhibitory pair in the ventral spinal cord comparable to the excitatory/inhibitory pair formed by glutamate & GABA in the brain. Interestingly, the two exitatory amino acids -- glutamic acid & aspartic acid -- are the two acidic amino acids found in proteins, insofar as both have two carboxyl groups rather than one. (ref.)
Glutamate: (Yes, the same stuff that's in MSG) - transmitter used to drive fast excitatory synapses. Too much and it becomes 'excitotoxic' and can cause neuron cell death.
Histamine:
associated with wakefulness. In the hypothalamus, histamine is released according to a circadian rhythm. Peak releases of histamine occur during the night together with enhanced locomotion. These observations have led to the idea that brain histamine is implicated in arousal mechanisms and that, in the posterior hypothalamus, histamine released from its neurons contributes to wakefullness and increased locomotion in the night. ... Brain histamine also seems to be involved in memory processes. Central administration of H3 agonists deteriorates, while H3 antagonists improve short-term memory. (ref.)
Epinephrine:
Epinephrine is both a hormone and a neurotransmitter. It's more commonly known as adrenaline. The term "adrenergic" means "epinephrine-using", and is used to describe a class of G-protein coupled receptors that respond to epinephrine. The principal catecholamines are norepinephrine, epinephrine and dopamine. These compounds are formed from phenylalanine and tyrosine. Tyrosine is produced in the liver from phenylalanine through the action of phenylalanine hydroxylase. The tyrosine is then transported to catecholamine-secreting neurons where a series of reactions convert it to dopamine, to norepinephrine and finally to epinephrine.
Catecholamines exhibit peripheral nervous system excitatory and inhibitory effects as well as actions in the CNS such as respiratory stimulation and an increase in psychomotor activity. The excitatory effects are exerted upon smooth muscle cells of the vessels that supply blood to the skin and mucous membranes. Cardiac function is also subject to excitatory effects, which lead to an increase in heart rate and in the force of contraction. Inhibitory effects, by contrast, are exerted upon smooth muscle cells in the wall of the gut, the bronchial tree of the lungs, and the vessels that supply blood to skeletal muscle.
In addition to their effects as neurotransmitters, norepinephrine and epinephrine can influence the rate of metabolism. This influence works both by modulating endocrine function such as insulin secretion and by increasing the rate of glycogenolysis and fatty acid mobilization.
The catecholamines bind to two different classes of receptors termed the a- and b-adrenergic receptors. The catecholamines therefore are also known as adrenergic neurotransmitters; neurons that secrete them are adrenergic neurons. Norepinephrine-secreting neurons are noradrenergic. The adrenergic receptors are classical serpentine receptors that couple to intracellular G-proteins. Some of the norepinephrine released from presynaptic noradrenergic neurons recycled in the presynaptic neuron by a reuptake mechanism.
(ref.)
Norepinephrine:
Also known as noradrenaline, this is widely encountered throughout the nervous system. In the central nervous system, the function of norepinephrine usually complements that of ACh (Acetylcholine); thus norepinephrine acts in the general direction of arousal, and ACh tends toward restorative functions. Norepinephrine dilates the pupil of the eye, strengthens and speeds the heartbeat, and inhibits processes of digestion, all under the heading of what has been called the “fight or flight” response; it also stimulates the adrenal glands to release epinephrine and the liver to release large quantities of glucose, which make more energy available to the muscles for action. Several drugs that work by means of the norepinephrine system are useful in asthma; these are known as the beta-agonists, because they are targeted to a specific group of “beta” receptors in the bronchial muscles, where they relieve constriction.
...
The neurons in the brain that contain norepinephrine cluster in a small region of the brainstem; their axons project to the hypothalamus, the cerebellum, and even the forebrain, a good 10 to 15 centimeters away. Norepinephrine is associated not only with alertness and arousal but also with the dreaming phase of sleep and, by way of the hypothalamus and the limbic system, with the regulation of mood. For instance, a number of studies point to depleted levels of norepinephrine at brain synapses, or a reduced ability of receptors to use it, as a factor in depression. Not that this amounts to a scientific formula that a normal brain minus some amount of norepinephrine equals a depressed mind; such formulas are far too coarse—particularly in an area like the basis of mood, where any number of elements may interact. Moreover, some of the factors that are undoubtedly important for mood are unquantifiable, invisible, and perhaps irreproducible for laboratory study. One point of wide consensus, however, is that depression can be helped by two classes of drugs: one class blocks an enzyme that would normally break down norepinephrine in the synaptic cleft, and the other slows the reuptake of norepinephrine into the presynaptic cell.
...
It is by means of different receptors that norepinephrine, which supplies blood vessels in both skeletal muscle and skin, can cause constriction in the vessels of the skin and dilation in those of muscle. (ref.)
Dopamine:
Dopamine is chemically similar to serotonin and norepinephrine, and it overlaps with them in several biological functions. Formed, like serotonin, from an amino acid, dopamine is actually a precursor to norepinephrine—the same compound except for one different chemical bond—and a wide-ranging neurotransmitter in its own right. In many systems, dopamine acts as an “off” switch: it halts the release of prolactin (which is responsible for the function of the mammary glands), inhibits some cells of the olfactory tract, and also shuts off some of the action of autonomic nerve cells (although this function is not well understood).
Elsewhere in the nervous system, dopamine is important for the control of movement; the degeneration of dopamine-using neurons in a portion of the midbrain leads to Parkinson's disease. A patient with this condition finds it difficult to initiate movement and also to stop, and to manage associated actions such as swinging the arms while walking. A slow tremor of the hands and head, present when the patient is at rest but not during movement, is probably what gave the illness its original name of “the shaking palsy.” Although the progress of the disease cannot be halted, the symptoms of Parkinson's disease can be effectively controlled in most patients by treatment with L-dopa. (This is not the actual transmitter itself but a precursor, a molecule that has the ability to pass through the blood-brain barrier and from which the brain can form dopamine.) Because dopaminergic neurons are also well distributed in the limbic system, we would expect some role for this neurotransmitter in the creation of mood—and, indeed, evidence to this effect is accumulating. Most striking are the signs that a relative increase in dopamine activity in the frontal cortex may provide the biochemical basis for schizophrenia. (ref.)
Cocaine binds to Dopamine receptors.(ref.)
Adenosine: of ATP fame.
Prolonged increased neural activity in the brain's arousal centers triggers the release of adenosine, which in turn slows down neural activity in the arousal center areas. Because the arousal centers control activity throughout the entire brain, the process expands outward and causes neural activity to slow down everywhere in the brain [leading to drowsiness]..."We knew that coffee kept us awake," Dr. Greene said. "Now we know why: Coffee and tea are blocking the link between the prolonged neural activity of waking and increased levels of adenosine in cells, which is why they prevent us from getting drowsy." (ref.)
Nitric oxide: The molecule nitric oxide fills a critical role in diverse tissues of the body, from the lining of blood vessels to the cerebellum, but its identity as a messenger of anything at all was completely unsuspected for a long time. For one thing, the compound—a single atom of nitrogen joined to one atom of oxygen—was very unstable, existing only for a matter of seconds. What could it be doing in the body? It was the well-known effect of nitroglycerin on intense chest pains that first put investigators on the trail of nitric oxide as a messenger molecule. Nitroglycerin works in remarkably small doses to dilate the blood vessels and relieve chest pain. Pharmacologists already knew that nitric oxide was the active ingredient formed by the body from nitroglycerin. But what became clear only in the late 1980s was that nitric oxide was the very substance being sought independently in cardiovascular research as a “relaxing factor” that works in tandem with the neurotransmitter ACh in the lining of blood vessels.
It soon emerged that nitric oxide does not bring about this effect alone; rather it stimulates the production of a second messenger, cyclic GMP (cyclic guanosine monophosphate). As for its origin, nitric oxide is formed by the action of an enzyme from the amino acid arginine; another acid, citrulline, is given off as a by-product. One of the reasons nitric oxide has been so difficult to find in the body is that it is so shortlived (its half-life is five seconds). But the citrulline that is produced at the same time does remain in the system and it can be measured, providing a clue to the evanescent presence of nitric oxide.
At this point in the inquiry, brain researchers began to take an active interest. Solomon Snyder, director of the neuroscience department at Johns Hopkins Medical School, was intrigued by the actions of nitric oxide, and especially by their extraordinary rapidity. He felt sure a system as remarkable as that of nitric oxide could not have developed only for use in blood vessels—it must also be at work somewhere in the brain. Snyder's research team used as their starting point the established fact that when the neurotransmitter glutamate binds to receptor sites, the calcium channels open and a great amount of cyclic GMP is produced. Once the investigators knew what to look for, they could follow two lines of evidence simultaneously: levels of citrulline and levels of cyclic GMP, both of which indicate the action of nitric oxide. Sure enough, stimulating the glutamate receptors in the cerebellum tripled the levels of citrulline and increased the levels of GMP almost tenfold. (Conversely, when the cells were treated with a drug that inhibits the nitric oxide-forming enzyme, no cyclic GMP was produced, even when the glutamate receptors were activated—a check on cause and effect that confirmed the researchers' hunch.)
As another piece of evidence, these great increases in cyclic GMP production all took place within a few seconds. This was a remarkably swift reaction, well within the time frame of some of the more brisk neurotransmitters. It appears that the nitric oxide-forming enzyme is switched on as soon as the calcium channels open, and it begins at once to produce nitric oxide at full speed, with some help from calmodulin, a calcium-binding protein. Thus, nitric oxide is indeed at work in the brain, and it is associated with one of the most important excitatory neurotransmitters — glutamate.
Intriguingly, nitric oxide is neither a transmitter nor a second messenger but truly a different type of messenger between cells. By mapping the areas of the brain where the nitric oxide–forming enzyme tends to concentrate, investigators are beginning to understand more about the action of this substance. Nitric oxide–forming enzyme is found in high concentrations in the cerebellum, which is largely involved in movement, and in the olfactory bulb; it also appears in the pituitary gland (the producer of many hormones), in sections of the eye, and in the intestine (where it may turn out to be the primary agent of muscle relaxation). One of the most exciting of recent results is the finding that certain arteries in the brain contain the enzyme not only in their interior lining but, more unusually, in the neurons that supply the outer layer—the very arteries and nerves recently shown to be involved in migraine headaches. These observations are already being applied in the rapid development of drugs to act at this site in the nitric oxide system, and may soon bring millions of migraine sufferers a new prospect of relief. (ref.)
---
Many other neurotransmitters are derived from precursor proteins, the so-called peptide neurotransmitters. As many as 50 different peptides have been shown to exert their effects on neural cell function. Several of these peptide transmitters are derived from the larger protein pre-opiomelanocortin (POMC). Neuropeptides are responsible for mediating sensory and emotional responses including hunger, thirst, sex drive, pleasure and pain. (see Peptide Hormones and Receptors for more info).
Comments